While the software industry is currently grappling with ideas of complexity and resilience, there has been very little in the way of concrete actions or activities that software engineers can use to actually design systems. Residuality theory answers this need and draws on complexity science and the history of software engineering to propose a new set of design techniques that make it possible to integrate these two fields. It does this at the expense of two of the most important concepts in software design: processes and components. (Not a member? Download a complimentary copy.)
Executive Update
“There Is No Spoon”: Residuality Theory & Rethinking Software Engineering
Posted July 20, 2020 | Technology |
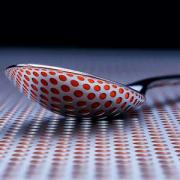
Don’t have a login?
Make one! It’s free and gives you access to all Cutter research.