Jason Radel explores the application of a digital twin framework for the ingestion, application, and visualization of digital twins and the integration of light detection and ranging data, photographs and scans, and other engineering documents. The article includes case studies from the energy and defense sectors, demonstrating how such an approach can be used in managing digital twins in different industries.
Article
Exploring Digital Twin Potential for Energy & Defense
By Jason Radel
Posted April 30, 2023 | Technology | Amplify
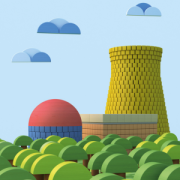
Don’t have a login?
Make one! It’s free and gives you access to all Cutter research.